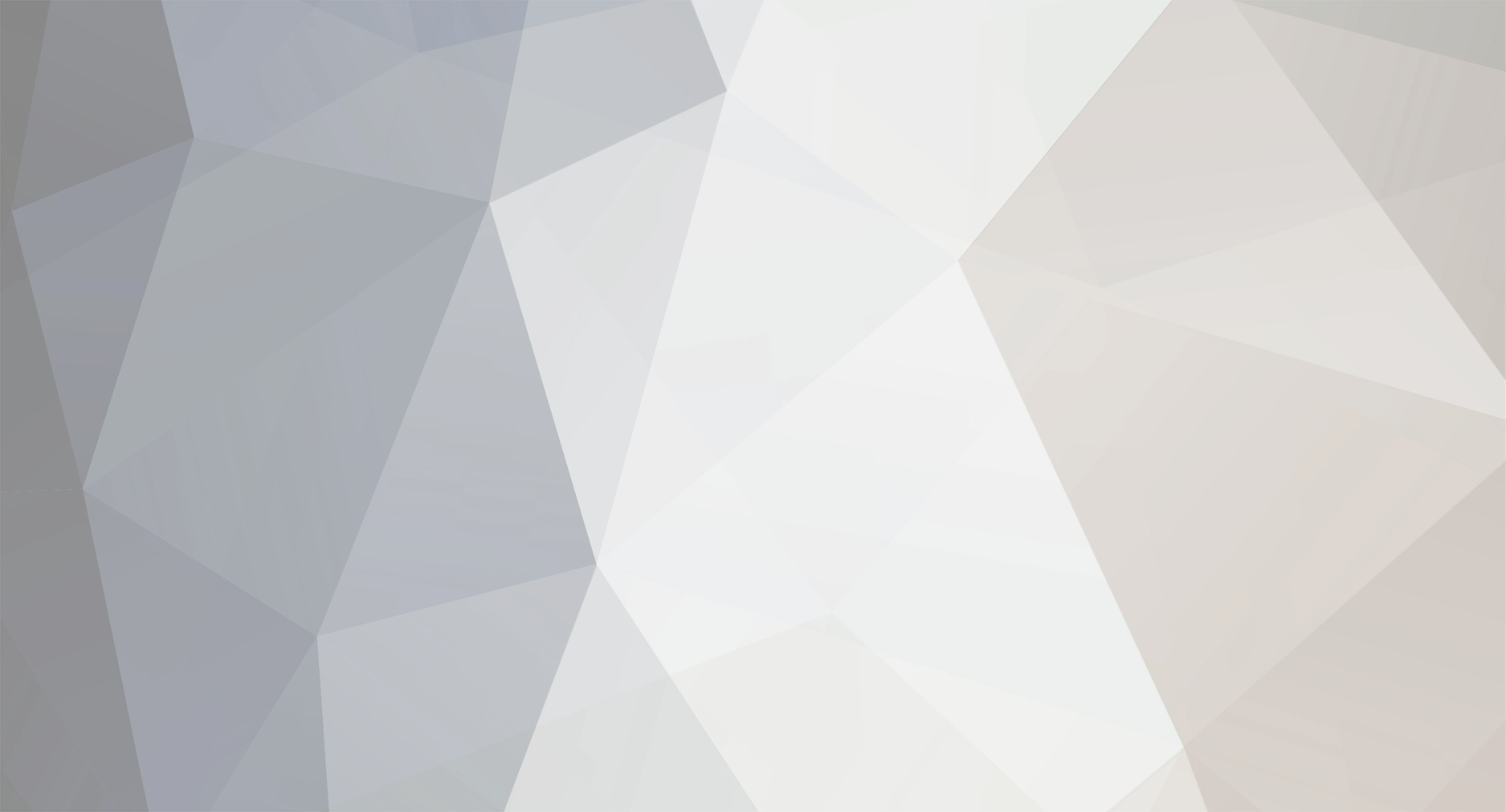
Tony K
Members-
Posts
294 -
Joined
-
Last visited
Never
Content Type
Profiles
Forums
Blogs
Gallery
Calendar
Downloads
Everything posted by Tony K
-
"Case B. The theory doesn't meet (1), or possibly (2), but it does meet (3). This is the realm of "the rule of thumb" -- it works, but no one knows why." Chaos theory? There is a butterfly flapping its wings in the Amazon which is upsetting our results. Seriously, to prove a theory we need reliable and repeatable results, which depend on reliable measurable inputs. So what can we measure? 1. Power input simply done with a wattmeter. 2. Efflux velocity. Difficult to measure accurately. As Erfolg wrote, there is a velocity gradient across the duct. There may be turbulence. The flow might not be axial. 3. Static thrust. This should be measurable to a reasonable degree of accuracy. Given the thrust and the inlet/outlet dimensions we can calculate the theoretical values of the other factors. The theoretical values can be "massaged" by making some plausible guesses ( eg.the effect of triangular intakes or bifurcated ducts) until an overall theory emerges. It appears to me that a reliable thrust measurement is essential. So, Richard, how confident are you that your thrust measurement figures are accurate? Do you have a picture or diagram of the measuring apparatus?
-
Posted by Richard Sharman on 28/10/2011 23:03:13: I still think your acceptance of Scharnhorst's 85% is mistaken... I would be surprised if they were 85% efficient. I suspect this figure is not right for the case we are discussing, and we should be trying to find it out, rather than assuming it. I agree, 85% is very optimistic. On the numbers I posted, yes, I did post 350w and 42m/sec but they were not with the plane is the same setup. My misunderstanding. One reason I am not keen on your average velocity derivation is because it is not practical to measure average velocity, so we have not way of making an independent check. I do not understand your reluctance to accept this. We can calculate the velocity ratio by rearranging vi.Ai = ve.Ae. So if we know the velocity at one end, we can simply calculate the velocity at the other end and the average. The working you have given (first post of 28/10) is (interestingly) independent of the plane's speed, so applies to the static case as well as the dynamic (your argument, I believe?). Yes that is my argument. I believe the motion impulse calculations show that, theoretically, static thrust and dynamic thrust (at any speed) are equal. Now, a quick look at your test figures: "test1: ve=47m/sec, T=6.1N" Mass flow = ve x Ae x rho = 47 x 0.002463 x 1,2 = 0,139 kg/s. From the velocity ratio, vi = 31,6 m/s, so dv = 15,4. Thrust = mdot.dv = 0,139 x 15,4 = 2,14N ps, "(in the nicest possible way, I hope)" If this discussion was anything other than polite and constructive, I would not take part. Edited By Tony K on 30/10/2011 08:09:34 Edited By Tony K on 30/10/2011 08:11:13
-
On the question of Scharnhorst using the kinetic energy equation (1/2 mv^2) to calculate impulse gain or loss. The reason I find this invalid is because there is no delta or varying property in that equation so it can not represent an increase or decrease, only a steady state. I know that combining the equations for inlet and exhaust gives a delta v^2 but this only tells you the difference between the steady state at the inlet and the steady state at the exit, ie. the changes within the system boundaries. There are simpler ways of calculating that. The (what I believe is) correct equation, I = m.dv, gives you the actual gain or loss because a property has changed. It tells you how the "black box" reacts in its environment. For example, in a previous post I suggested that the intake velocity is 6m/s higher than free stream velocity so there is something changing outside of the black box but being caused to change by a mechanism inside the box. That something is a change of momentum, in this case an impulse gain, and by comparing the momentum at the inlet and the momentum of free air (air unaffected by the mechanism) it can be given a value. This, I believe, is a fundamental flaw in Scharnhorst's theory but I know Richard will not agree with me! Edited By Tony K on 28/10/2011 11:32:13
-
Richard, the only assumption I have made is that Scharnhorst is correct when he writes (on page 12), "...indicates that the ducted fan (generally) can achieve its best effiency of approx. 82 - 85%..." On the 10th. Oct. you wrote, "Actual consumption as measured by battery depletion is 350 Watts". On the 19th. Oct. you wrote, "Consequently, I claim the value given above (42m/s) for average flying speed is reliable, at least to the level of accuracy we are working here". I did not want to clutter this thread with unecessary calculations but this is how I arrived at the previous figures. As a starting figure I used 296 W as useful power output. I want to know how much mass flow and thrust can be obtained from that power.So I need to know the velocity at a point where I know the cross sectional area. Starting at the inlet, Ai = 0,003667m^2. Vi is unknown, I will call it "u". The exit area, Ae = 0,002463m^2, so from Ve/Vi = Ai/Ae the exit velocity is 1,4888u (I will use1,5). I now know that the average cross section Aav = 0;003065 m^2 and the average velocity Vav = 1,2444u m/s (I will use 1,25). The mass flow can be obtained from:- density x Aav x Vav. mdot = 1,2 x 0,003 x 1,25u = 0,0045u Moving on to the thrust, T = mdot x dV or mdot.Ve - mdot.Vi. T = (0,0045u x 1,5u) - (0,0045u x u) = 0,00225u^2. Power can be calculated from P = thrust x average velocity. P = 0,00225u^2 x 1,25u = 0,00281u^3. Using the assumed power figure of 296W I can find the value of u. u = cube root of (296/0,00281) = 47,2m/s. I rounded this up to 48m/s and ran it through the calculations:- Vi = 48m/s. Ve = 48 x 1,4888 = 71,46. mdot = 0,2112 kg/s. Thrust = 4,955N These calculations can be repeated for any power output and will give a theoretical thrust based on the sizes of the inlet and outlet. Quote, "This assumption is not warranted here unless you have some independent way of obtaining it, but you don't show it". I hope it is shown to your satisfaction. T.K. Edited By Tony K on 28/10/2011 09:54:59
-
On to the K/S (K.Scharnhorst or Kinetic energy/S) equation, 1/2 mdot.v^2. Combining the input and exit gives 1/2 mdot(ve^2 - vi^2) which gives a result in Nm/s = Joules/s = Watts, ie the power output. This is not the impulse (change in momentum). Working through the figures:- Power = (0,2112 (71,46^2 x 48^2))/2 = 296 W. But we can also use thrust times average velocity to achieve the same result:- TVav = T((Ve + Vi)/2) = 4,9547((71,46+48)/2) = 296 W. All things considered, I think the Scharnhorst paper is of very little use for designing or refining a ducted fan system. Your comments invited, T.K.
-
Let me present the following for your consideration. We have an EDF aircraft which draws 350W of battery power to fly at 42 m/s airspeed (Vo). Assuming an effiency (power out/power in) of 85% we have 296W being used for useful work. By calculation, 296W gives a thrust = 4,955 N and the mass flow = 0,2112 kg/s. The inlet area = 0,003667 m^2 and the outlet area = 0,002463 m^2 so the inlet velocity (Vi) = 48 m/s and the outlet velocity (Ve) = 71,46 m/s. Now look at the impulse calculations. Impulse is change in momentum, so I=m.delta v. Impulse gain at the inlet = (Vi - Vo)m and impulse loss at the outlet = (Ve - Vo)m. So pgain = (48 - 42)0,21112 = 1,2672 N and ploss = (71,46 - 42)0,2112 = 6,22195 N. ploss - pgain = 4,9547 N. This corresponds to the thrust figure above. Now the static case, Vo = 0, with the same mass flow. pgain = (48 - 0)0,2112 = 10,1376 N and ploss = (71,46 - 0)0,2112 = 15,0923 ploss - pgain = 4,9547 N Therefore, theoretically, static thrust equals dynamic thrust for the same mass flow. Edited By Tony K on 27/10/2011 10:45:37 Edited By Tony K on 27/10/2011 10:47:15
-
Posted by Keith Simmons on 25/10/2011 13:36:45: I think the EDF force works at both ends. The intake as a suction force which tries to move the fan forward, and the exhaust as thrust against static air. In dynamic mode (flying) The suction in front of the EDF would be less, so less resistance for the fans and therefore an increase in speed. Keith, in non mathematical terms, there is less "pull" in front of the fan in the dynamic case because incoming air is already moving but there is also less "push" behind the fan because that which is being pushed against is also already moving. Richard, Erfolg, that is the basis for my argument above, "I would suggest that the impulse gain in the static case is balanced by the impulse loss of ejecting the "exhaust" into static air instead of air moving at free stream velocity. Therefore these two factors (outside of the system boundaries) cancel out." I am of the opinion that this is a flaw in Scharnhorst's static model. Furthermore, as "impulse" is change in momentum and we assume (questionably) that in the dynamic case vi equals the flying speed, where is the change in momentum at the intake? The velocity of the mass of air about to enter the inlet has the same velocity after it has entered (ignoring lip geometry). This suggests to me that the impulse gain is zero in the dynamic case and has a positive value in the static case. What do you think?
-
Quote, "Where is the energy coming from to create this impulse gain ? - only from the ducted fan system, since that is the only energy source around. So the system has an energy loss exactly equal to this unknown inlet energy gain, and the two cancel out." I would suggest that the impulse gain in the static case is balanced by the impulse loss of ejecting the "exhaust" into static air instead of air moving at free stream velocity. Therefore these two factors (outside of the system boundaries) cancel out. The calculations can then use measurable properties from inside the system boundaries.
-
To expand on my doubts about the dynamic calculation and thinking about Richard's question on page 1, "where are all the watts going?" The dynamic thrust calculation is based on one fundamental assumption, that Vi = flying speed but is that assumption correct? Richard's Hawk uses 350W from the battery to push it through the air at 42 m/s but the calculations suggest that the power output is considerably less than 350W. Perhaps, then, the calculated thrust figure is simply too low. Let's say that Vi is 48m/s so the intake is drawing air faster than the 42m/s free stream. One could visualise this as a partially static condition. Running this Vi figure through the calculations gives a thrust of 4,9N and a power output of 296W. Adjust this for 15% losses and you have 350W. W/N = 71. Richard, your static "Test 2" figures show a thrust of 10,5N for an input of 791W, 75W/N. It would be interesting to see what thrust you get with 350W input. I suspect it would be close to 4,9.
-
I must admit I am struggling with this. Quote, "...but in the static case it is less clear what the inlet size is because air is being drawn from a much wider area, inevitably at lower speed". I take this to mean that at some point upstream of the system boundary V=0. By the same argument, at some point downstream of the system boundary Ve reverts to zero. Shouldn't we also set Ve to zero and ignore it? Also, quote, "...dv is now equal to the exit velocity ve of the air stream at the nozzle since the airstream has been accelerated from 0 to ve...." A system which has an exit velocity and no inlet velocity is a rocket. Looking at the thrust curves it appears that the pink curve ( I hope you don't mind if I do not refer to it as the static curve ) has a Y value about twice that of the dynamic curve (I also have some doubts about this). Is this because half the equation is missing? Beause of the questions above and Dizz's observationsI can not accept Scharnhorst's theory as realistic.
-
I still have a problem with the assumption vi=0. As Dizz stated earlier, "6. In the static case intake air has to be moving into the duct: the mass of air being expelled has to be the same as the air entering the duct ..." I think in the static case we need to look outside of the system (the system being that which is between the inlet and outlet apertures). In order to draw surrounding air into the inlet there must be work done to the surrounding air, it is given some energy. That energy comes from power consuption of the fan. Dynamically the free stream velocity is very close to inlet velocity so does not need any work done to it. So for the static inlet flow to equal dynamic we need more power. If more power is not available the static inlet flow will be less than dynamic. I think this is Keith Simmons' point.
-
After a quick google I have found quoted figures for the Memotec mini fan powered by a Mega 16-15-2F of 10N thrust drawing 55A from a 3s lipo. Using 3,5 V/cell the power consumption is 577,5 W. That means 57 Watts per Newton thrust. Now I don't know how these figures were obtained, static/dynamic, with/without ducting, etc. or whether they are even accurate. Richard's Hawk is using 350 W to provide 3,9 N, that is 89 Watts per Newton - a considerable difference.
-
Posted by Stefan Hafner on 21/10/2011 12:27:45: This is a lecture slide from at uni, where it can clearly be seen that when going faster that the effective angle of attack is lower, and as the prop can be treated as a wing at lower angle of attack the trust (lift) will be less. this example is for a propeller but the same princlple will apply to a fan. Hence constant speed props on upmarket light aircraft. For fixed pitch props/fans surely the RPM will increase until the resistance matches the available motor torque? Posted by Erfolg on 21/10/2011 13:09:10: I think the optima, fanjet and the ducted engines seen on blimps, have more in common in what interests us modellers than the exotic, however much they excite us (well at least me). Agreed. Better to leave supersonic aircraft out of this discussion.
-
Richard, do you have any static figures for your Hawk?
-
Erfolg, it was I who suggested that pressure is constant. By that I mean air pressure, which is 1 atm (about 101 kPa). This is not the same as pressure head (units of length) which can change according to Bernoulli. As regards the collapsing ducts, take the duct downstream of the fan. Air leaves the exit faster than it passes through the fan and part of the net trust or force is gained from this acceleration. Now imagine a cross section anywhere in the duct. Its job is to receive air from the previous cross section and squeeze it (exert a force on it) so that it fits into the next cross section. This squeezing does not increase the air density (or air pressure) because it is able to escape downwind if it moves a bit faster but the sqeezed slice of air is also exerting an equal and opposite force on the duct wall. If this force overcomes the strength of the wall, plus the atmospheric pressure outside, the wall will fail.
-
Richard, I see now that Scharnhorst is using the kinetic energy equation, 1/2 (mv^2), but by using mass flow instead of mass he has introduced the time factor which gives a result in Joules/sec and Watts = J/s. The other equation gives a result in (Nm)/s = J/s, so as you say they are algebraically equvalent. I would be interested in seeing your analysis of the static condition although I think it is the free stream velocity which should be zero not vi. If vi = 0 there is no flow at all through the system. Stefan, this thread was created after "Rickenbangler's" question about inlet duct size and Richard has set himself the task of analysisng the theory with the hope of applying it to practice. I am sure that various ducting principles will come up at some stage of the discussion. Be careful about using the term pressure, air is considered to be incompressible at our velocities. TK
-
OK Richard, let's do some sums. Using your first figures- vi=46, ve=68.5, dv=22.5, mdot=0.21 (I prefer to use "mdot" for mass flow), gives a thrust T=dv x mdot = 4,725 N. KS equation Pf = (1/2)*M*(ve^2 - vi^2) = (1/2)*0,21*(68,5^2 - 46^2) = 270,5 W. My equation Pf = T x Vav = 4,725 x 57,25 = 270,5 W. Therefore Ploss - Pgain = T x Vav.
-
Richard, thank you for your comments on the other thread, I will be following this one with interest. Paragraph 5 in your Scharnhorst summary is confusing. Shouldn't Pgain be that which you get out of the system and Ploss be what you have to put in.? The way it is written will result in a negative Pfan value. Anyway is this impulse calculation necessary? As the thrust is produced by the whole system, from intake to exit, wouldn't the average velocity (1/2 Ve - Vi) be the factor in the equation. ie. P=T x Vaverage. Using your airspeed figure of 46 m/s as Vi, the Ve is 68,5 m/s. So Vav is 57,25 m/s and dV is 22,5 m/s. The Qi value gives a mass flow (mdot) of 0,2 kg/s so net thrust = mdot x dV = 4,5N. P = thrust x velocity = 4,5 x 57,25 = 257 Watts. Using a fan efficiency of 85% we are now up to 321 Watts for the motor. By the same process an airspeed of 42 m/s gives a motor power of 253 Watts so about 28% of your 350 W is missing. This all assumes that Vi = airspeed. Is that assuption correct? Could there be some acceleration of the air in front of the intake?
-
Hello everybody, been lurking for many months but this is my first post. Although fluid mechanics is not my field, may I add a few thoughts here. As I see it, a ducted fan works by accelerating a mass of air rearwards and the reaction to this acceleration is what drives the model forwards. Therefore I believe it is the mass flow which should be considered. Mass flow is density times cross section area times velocity. As we are not adding or subtracting heat, I think we can ignore density. So as mass flow is constant through a closed system, the relationship of cross section area (A) and velocity (V) is the concern. Imagine the air immediately in front of the fan and the air immediately behind. A (FSA) is the same so V must also be the same. This means that air is accelerating in the upstream duct. If we call the velocity at the intake (model flying speed) V1 and the intake area A1, similarly the velocity at the fan V2 and the FSA A2, we get V1xA1 = V2xA2. The area of the intake should theoretically be A1 = V2xA2 divided by V1. So the intake area should be larger than the FSA. How much larger is in proportion to the difference between V1 and V2 (theoretically, fan pitch times RPM), ignoring intake and duct losses. Assuming the same EDF, for a relatively slow model V2/V1 is greater than for a fast model so the slower model needs proportionally larger intakes. I can not imagine a system where the intake area is smaller than the FSA as the incoming air would decelerate before being re-accelerated by the fan. Downstream of the fan is less important. The mass of air has already been accelerated so we just need to let it out without losing too much of the increased velocity. Constant cross section or small convergence should achieve this. I hope this makes sense.